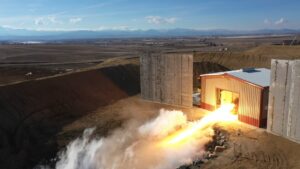
Colorado-based propulsion firm Ursa Major has announced that it has successfully flight-tested a long-range solid rocket motor for the Army. This particular program saw Ursa Major progress from concept and design to live-fire testing in under four months. Leveraging their innovative additive manufacturing techniques, agile manufacturing systems, and propulsion engineering expertise, they collaborated with Raytheon to achieve this rapid timeline.
Ursa Major’s manufacturing cell produces its own energetics on-site to power the motors and has developed multiple configurations for programs including the Navy’s Standard Missile platform. The company aims to revolutionize the solid rocket motor production process through increased use of additive manufacturing, bolstering the nation’s ability to replenish depleted weapon stocks like the Javelin, Stinger, and Guided Multiple Launch Rocket System (GMLRS). This approach also enables Ursa Major to rapidly switch production to other solid rocket motor models without significant retooling or added costs. Their Lynx strategy can 3D print approximately 50 small engine cases, up to 2.5 inches in diameter, within three days. This process can reduce costs associated with traditional manufacturing methods, such as machining. Additionally, it allows for swift design iterations and rapid changes as engineers only need to modify the digital model representing the part. As long as they adhere to predefined parameters, new parts can be produced in hours instead of weeks.
The defense industry has faced constraints due to limited solid rocket motor production pipelines in recent years, prompting multiple firms to explore new avenues. This program demonstrates Ursa Major’s ability to scale up solid rocket motor production to an industrial level and contribute to meeting U.S. defense needs.
In certain countries, engineers are investigating the use of 3D printing to deposit conductive materials for electrical circuits, create housings for revolutionary gallium nitride transmitters, and fabricate fins for guided artillery shells. Engineers are exploring novel designs for thermal improvements and lightweight structures, which were previously unattainable with traditional manufacturing methods. Ensuring consistent production integrity will be crucial in realizing this vision. The current method of building microscopic circuits involves material removal to create a circuit pathway. In contrast, 3D printing deposits only the necessary material to construct the electronic pathway. While circuits can already be printed using inkjet printers, the goal is to 3D print more complex circuits with the high resolution and performance of silicon.
3D printing is well-suited for the military-industrial complex. Military manufacturing often demands highly specific components, such as a specialized bolt for a particular aircraft with a limited production run and strict weight constraints. Even items produced in large quantities, like guns and ammunition, are relatively small compared to consumer products.
As a result, the standard economies of scale associated with mass manufacturing yield fewer benefits in the military-industrial complex. For instance, a nation ordering 500 missiles with 1,500 fins over five years may struggle to find a non-military metal stamping workshop willing to accommodate such a small order. This quantity might barely meet the minimum order quantity for most parts makers, and the manufacturer would typically expect multiple orders to offset the cost of tooling. However, with a machine capable of producing virtually any part within specific dimensions and materials with minimal tooling costs, the cost per missile fin could drop significantly, from hundreds of dollars to a more reasonable amount. The slower speed of 3D printing becomes less of an issue when the price differential is substantial.
3D printing also offers potential for scaling production. If a nation requires 100 missiles annually for inventory and training purposes, a few molds or tools may suffice. However, wartime demand could surge to 500 missiles per month, requiring a significant increase in production capacity. Scaling up production by a factor of 100 necessitates either a large stockpile of excess tooling or a lengthy lead time as the industry struggles to create and implement new tooling. This challenge, multiplied across various weapon systems, could delay the delivery of critical military equipment. 3D printers, on the other hand, can produce a wide range of components. A nation could maintain a fleet of 3D printers for civilian applications during peacetime, leveraging the military’s non-profitability to ensure competitiveness. In times of war, this fleet could quickly transition to producing military equipment without straining industrial and economic resources. 3D printing also holds promise for forward logistics. A recent example involves a marine who successfully repaired a mobile satellite system by 3D printing a replacement part. This technology could be valuable for forward operating bases with distant supply lines but reliable electricity, enabling the on-site production of specialized parts. In non-operational periods, these printers could be used for other purposes, such as producing novelty items for recreational use.